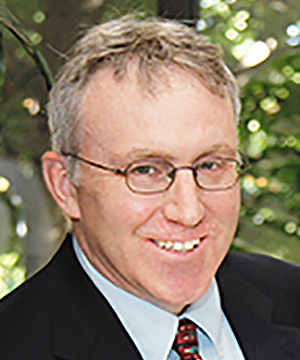
Todd D. Krauss
he/him/his
Jay Last Professor in Arts, Sciences & Engineering
Professor of Chemistry
Professor of Optics
PhD in Applied Physics, Cornell University, 1998
- Office Location
- 465 Hutchison Hall
- Telephone
- (585) 275-5093
- Web Address
- Website
Research Overview
Our research involves developing a fundamental understanding of the chemical, photophysical, and optical properties of nanometer scale materials. Unlike macroscopic materials, these objects have physical characteristics that are strong functions of their size and shape. Thus, nanometer scale materials have properties that can be easily controlled and manipulated. Currently, our investigations are focused on the synthesis and spectroscopic characterization of the chemical and physical properties of carbon nanotubes and semiconductor nanocrystals, and the application of these materials to solving important challenges in energy, photo-catalysis, quantum optics, and biomedicine. These studies are highly interdisciplinary, and lie at the interface between chemistry, physics, applied physics, and materials science.
We continue to be interested in making improvements to colloidal nanoparticle syntheses, as well as understanding the basic chemical mechanism for how molecular precursors react to form colloidal nanocrystals. In addition, we are also concerned with determining the relationship between structure and chemical composition and electronic and optical characteristics of individual carbon nanotubes, as well as colloidal nanoparticles doped with aliovalent impurity atoms (such as silver). These investigations are carried out using atomic electrostatic force microscopic in conjunction with single molecule optical spectroscopic methods (Figure 1).
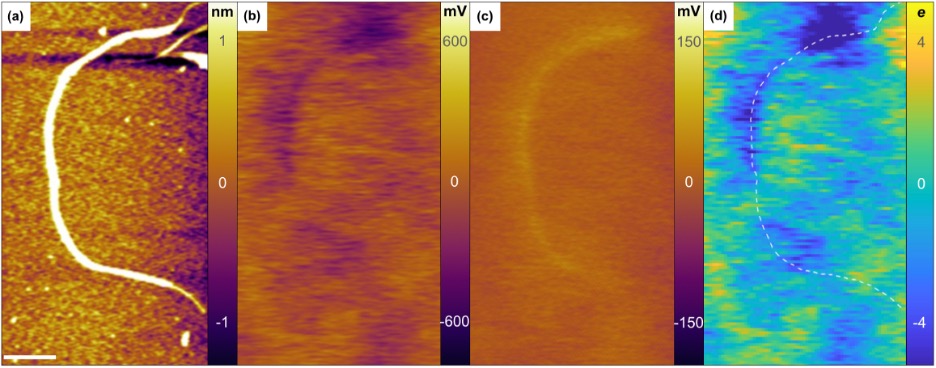
Leveraging the unique photophysical properties of colloidal nanocrystals to explore possible applications is also an important aspect of our research. In particular, solar energy can be converted to clean burning chemical fuels such as hydrogen using colloidal nanocrystals as the light harvesting element or photocatalyst. (This work is done in collaboration with Professors Bren and Matson from the University of Rochester.) Also, nanocrystals can be used in combination with inorganic or organic small molecule catalysts to make unique carbon-carbon bonds that are valuable to the pharmaceutical industry for making pharmaceuticals. (This latter work is done in collaboration with Professor Dan Weix from the University of Wisconsin.) Working with Handy Gelbard at the University of Rochester Medical Center, we are developing colloidal nanocrystals as biological mimics and combining them with super-resolution imaging techniques to better understand possible origins of disease including COVID long hauler syndrome.
In the last few years, we have developed a significant interest in the intersection of quantum optics and colloidal nanocrystals. Active projects include coupling nanoparticles and optical cavities to form hybrid light-matter states called molecular polaritons (Figure 2). We have a fundamental interest in understanding the photophysics of these exciton polaritons as well as using polaritons to perform chemical reactions that are not currently possible. This latter project is the focus of a new NSF Phase I Chemical Innovation Center (QuEST: Quantum Electronics for Selective Transformations).
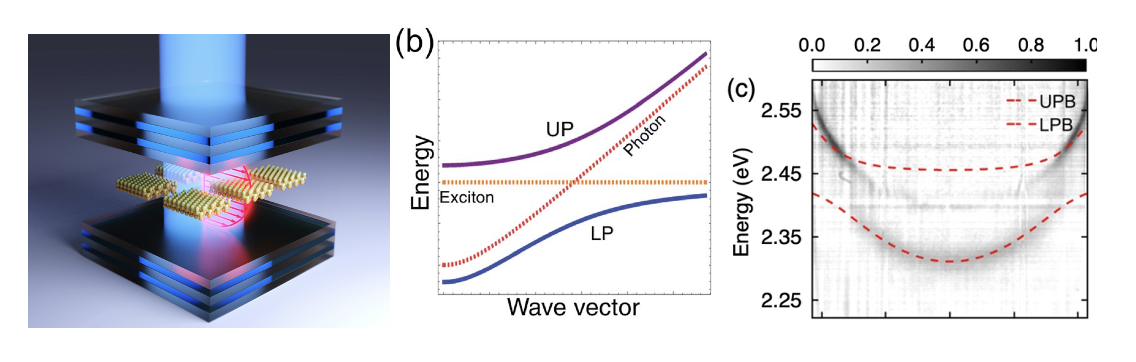
Research Interests
- Materials Chemistry and Physics of Colloidal Nanoscale Semiconductors
- Optics of nanoscale semiconductor materials
- Quantum optics and nanoscience
Selected Publications
- K. Widness, D. G. Enny, K. S. McFarlane-Connelly, M. T. Miedenbauer, T. D. Krauss, and D. J. Weix, “CdS Quantum Dots as Potent Photoreductants for Organic Chemistry Enabled by Auger Recombination,” J. Am. Chem. Soc. 144, 12229-12246, 2022.
- W. O’Neill and T. D. Krauss, “Synthetic Mechanisms in the Formation of SnTe Nanocrystals,” J. Am Chem. Soc., 144, 6251-6260, 2022.
- Qiu, A. Mandal, O. Morshed, M. T. Meidenbauer, W. Girten, P. Huo, A. N. Vamivakas, and T. D. Krauss, “Molecular Polaritons Generated from Strong Coupling between CdSe Nanoplatelets and a Dielectric Optical Cavity,” J. Phys. Chem. Lett., 12, 5030-5038, 2021.
- R. Amori, J. E. Rossi, B. J. Landi, and T. D. Krauss, “Defects Enable Dark Exciton Photoluminescence in Single-Walled Carbon Nanotubes,” J. Phys. Chem. C 122, 3599-3607, 2018.
- C. Frenette and T. D. Krauss, “Uncovering Active Precursors in Colloidal CdSe Quantum Dot Synthesis,” Nature Comm., 8, 2082, 2017.
- A. Caputo, L. C. Frenette, N. Zhao, K. L. Sowers, T. D. Krauss, and D. J. Weix, “General and Efficient C-C Bond Forming Photoredox Catalysis With Semiconductor Quantum Dots,” J. Am. Chem. Soc. 39, 4250-4253, 2017.
- Han, F. Qiu, R. Eisenberg, P. L. Holland, and T. D. Krauss, “Robust Photogeneration of H2 in Water Using Semiconductor Nanocrystals and a Nickel Catalyst,” Science 338 1321-3124, 2012.