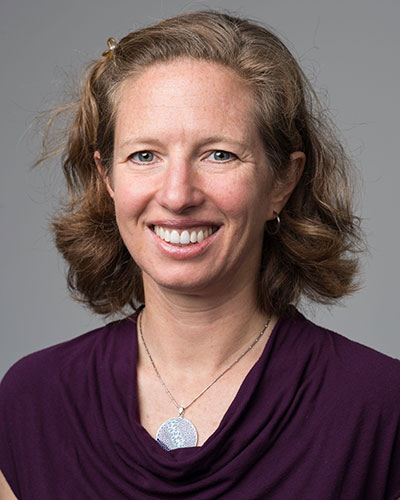
Farran Briggs
- Associate Professor, Brain and Cognitive Sciences, Neuroscience, Center for Visual Science
PhD, University of California, San Diego, 2003
6-8545 URMC
(585) 276-3736
farran_briggs@urmc.rochester.edu
Office Hours: By appointment
Research Overview
Critical to our comprehension of the brain is an understanding of how neuronal circuits, or the connections between neurons in the brain, underlie perception and behavior. The goals of the laboratory are to understand how neuronal circuits in the early visual system encode and process visual information and how spatial attention modulates these activities. There are two main projects that are currently underway in the laboratory. The first aims at understanding the mechanism by which visual attention modulates the activity of neurons and circuits in the early visual pathways. We have recently shown that attentional modulation of neurons in the primary visual cortex depends critically on the match between the feature selectivity of individual neurons and the features required for successful task completion. We continue to explore the mechanisms of attention at the granular and circuit level in order to develop a more comprehensive understanding of visual attention. The second project in the lab is designed to elucidate the functional role of the corticogeniculate feedback circuit in visual perception. We have shown that corticogeniculate neurons connecting primary visual cortex with the visual thalamus in the feedback direction are morphologically and physiologically diverse. Recently, we utilized an innovative combination of virus-mediated gene delivery and optogenetic techniques to show that corticogeniculate feedback controls the timing and precision of thalamic responses to incoming visual information. Ongoing experiments will further define how corticogeniculate feedback regulates the flow of information about distinct visual features in the environment.
Further details about the two main research projects in the lab
1. Neuronal Mechanisms of Attention
The phrase “visual attention” captures a broad range of phenomena. Most people consider visual attention to be an active cognitive process. For example, searching for a friend in a crowd involves attending to specific locations in space to locate the friend while also attending to specific visual features like the color of the clothing worn by the friend. When we study visual attention in the laboratory, we mainly study covert visual spatial attention, or the allocation of attention to a particular region of visual space that is away from the center of gaze. Covert visual attention is probably an important emergent property in animals, including humans, that care about facial expressions but are wary of making direct eye contact. We design visual attention tasks that involve shifts in the locus of covert spatial attention on different trials. Subjects are cued to attend to particular spatial locations and are rewarded for detecting subtle changes in visual stimuli at attended locations. In order to monitor attention behavior, the cue is occasionally invalid and subjects either miss the stimulus change all together, or react more slowly when detecting a stimulus change at an un-cued location. This type of cueing paradigm is called Posner cueing (Posner et al., 1980).
Covert spatial attention tasks have been utilized to study the impact of attention on neuronal activity throughout the visual system. Visual attention directed toward a stimulus overlapping the receptive fields of recorded neurons tends to increase the activity or firing rate of those neurons compared to conditions where the same stimulus is in the receptive field, but subjects are attending elsewhere. This attentional modulation of neuronal firing rate is characterized by an attention index, or the difference divided by the sum of neuronal activity across attention conditions (e.g. attending toward versus attending away from the stimulus in the receptive field). Interestingly, attentional modulation of neuronal firing rate scales along the visual hierarchy. Accordingly, attentional modulation of neuronal firing rate is modest in early visual structures such as the visual thalamus (LGN) and primary visual cortex (V1) and is more robust in higher visual cortical areas such as MT, V4 and FEF. However, in all of these visual structures, there is large variability in attentional modulation across individual neurons. This variability suggests that there may be rules governing whether and how much attention alters the activity of distinct sub-populations of neurons. Furthermore, attentional modulation of firing rate may not be the best readout of attention as the attention index is measured over long time scales (1-2 seconds) while attention modulation of actual neuronal activity may be highly dynamic. We previously discovered that attention can alter the efficacy of communication between neurons in the early visual pathways (Briggs et al., 2013) and we are interested in pursuing further explorations into the underlying biophysical, cellular, and circuit mechanisms by which attention alters neuronal activity.
Our most recent work illustrates the diversity of attention effects that can be observed in a single visual cortical area (Short et al., 2017). Specifically, we discovered that attentional modulation of V1 neurons is not uniform within the attended spatial region. Instead, attention selectively increases the firing rate of neurons that encode stimulus features that are important for successful completion of the task. These findings suggest that a general attention spotlight or spatial gain model of attention is too limited. Instead, models of attention must incorporate both spatial and feature attention components in order to accurately capture the variability in attentional modulation of neuronal activity, even for neurons responsive to the same attended spatial location. Future work will test this hypothesis explicitly by recording from the same neuronal populations while subjects perform multiple attention tasks involving detection of different visual stimulus features.
2. Functional Role of Corticogeniculate Feedback in Vision
In mammals, all conscious visual information traverses a path from the retina through the visual thalamus (called the lateral geniculate nucleus or LGN) to the primary visual cortex (V1). Accordingly, in just a handful of synapses and in about 50 milliseconds, information about a visual stimulus in the world can reach the cortex. In highly visual mammals such as humans, visual information traversing this path is segregated into three parallel processing streams. By separating out visual information that is most relevant for processing motion, form/acuity, and color, signals can be relayed in parallel for increased processing speed. Even though parallel stream segregation is a hallmark of the early visual pathways in highly visual mammals, we are not aware of this separation of visual information because signals from the parallel streams are combined in the visual cortex. We tend to think about visual information encoding as a feedforward process in which information progresses from lower visual areas, such as the LGN and V1, to visual cortical areas higher up in the processing hierarchy. However, the circuits of the visual system include many feedback projections in addition to the more commonly studied feedforward projections.
The first feedback circuit in the visual system is the corticogeniculate circuit connecting V1 to the LGN in the feedback direction. Corticogeniculate circuits are actually a subset of the larger family of corticothalamic circuits that link primary sensory cortex with the sensory thalamus in the feedback direction. As such, corticogeniculate circuits, and corticothalamic circuits in general, are thought to play an important role in regulating the feedforward flow of sensory information. In spite of many decades of study, the functional role of corticothalamic feedback in sensory perception has remained a fundamental mystery in systems neuroscience. Part of the difficulty in defining the functional role of corticothalamic feedback is due to the fact that these feedback circuits appear to merely modulate, rather than drive, changes in thalamic responses. In the visual system, corticogeniculate inputs onto LGN relay neurons far outnumber retinal inputs onto LGN relay neurons. However, LGN relay neurons have receptive field properties that mimic their retinal and not their cortical inputs. Are LGN relay neurons simply “relaying” (as their name would imply) retinal signals to the cortex, or is there some purpose for having a layover in the thalamus, midway between the retina and the cortex?
We have been dedicated to cracking the mystery of corticogeniculate feedback for many years. We previously discovered that corticogeniculate feedback is made up of multiple morphologically and physiologically distinct cell types (see Briggs et al., 2016; Briggs & Usrey, 2005, 2007, 2009). Interestingly, these distinct cell types map precisely onto the distinct feedforward streams that are the hallmark of the early visual pathways. We hypothesized that corticogeniculate feedback is carrying stream-specific information from V1 back to the LGN. But to what purpose?
Recently, we utilized an innovative combination of virus-mediated gene delivery and optogenetics to selectively and reversibly control the activity of corticogeniculate neurons in vivo. We discovered that enhancing corticogeniculate feedback dramatically reduced the response latency of LGN relay neurons to incoming visual inputs (Hasse & Briggs, 2017). In addition to responding more quickly to visual inputs, LGN neurons also responded more precisely and their receptive fields narrowed. Interestingly, we did not observe any changes in the tuning properties of LGN neurons with enhanced corticogeniculate feedback. Our results therefore confirm that corticogeniculate feedback is not responsible for driving the response properties of LGN neurons. Instead, corticogeniculate feedback controls the timing and precision of LGN responses to incoming visual information. We believe these findings will generalize to corticothalamic circuits across sensory modalities and perhaps extend to feedback circuits throughout the brain. Future research is aimed at understanding whether and how corticogeniculate feedback controls the timing of LGN responses in a stream-specific manner.
Featured Publications
- Hasse, J.M. & Briggs, F. (2017) Corticogeniculate feedback sharpens the temporal precision and spatial resolution of visual signals in the ferret. PNAS 114(30): E6222-E6230
- Hembrook-Short, J.R., Mock, V.L., Briggs, F. (2017) Attentional modulation of neuronal activity depends on neuronal feature selectivity. Current Biology 27(13): 1878-1887
- Bragg, E.M., Fairless, E.A., Liu, S., Briggs, F. (2017) Morphology of visual sector thalamic reticular neurons in the macaque monkey suggests retinotopically-specialized, parallel stream-mixed input to the lateral geniculate nucleus. J. Comparative Neurology 525(5): 1273-1290
Selected Publications
Selected Publications are available in NCBI MyBibliography
Research Collaborators
Dr. Mike Hasse, Post-doctoral Fellow, Neuroscience
Research Support
The National Eye Institute provides support for this research.